Volume 37, Issue 2 ⦁ Pages: 311-330
Abstract
Most research involving alcohol and cancer concerns the relationship between alcohol consumption and cancer risk and the mechanisms of carcinogenesis. This review relates the amount and duration of alcohol intake in humans and in animal models of cancer to tumor growth, angiogenesis, invasion, metastasis, immune response, and host survival in specific types and subtypes of cancer. Research on the influence of alcohol drinking on human cancer patients is limited. Although there is more information in animal models of cancer, many aspects still are ill defined. More research is needed to define the mechanisms that underlie the role of alcohol on cancer progression in both animals and humans. Activation of the immune system can play a positive role in keeping cancer under control, but this also can facilitate cancer progression. Additionally, a functional immune system is required for cancer patients to achieve an optimal response to conventional chemotherapy. Insight into the underlying mechanisms of these interactions could lead to effective immunotherapeutic approaches to treat alcoholics with cancer. Defining the epigenetic mechanisms that modulate cancer progression also has great potential for the development of new treatment options not only for treating alcoholics with cancer but also for treating other alcohol-induced diseases.
Alcohol use and abuse have been implicated as etiological factors in the genesis of an increasing number of cancer types in both men and women. In 2012, the International Agency for Research on Cancer (IARC) listed both beverage alcohol (i.e., ethanol) and its major metabolite, acetaldehyde, as tumor-inducing substances (i.e., carcinogens) in humans. The most recent worldwide statistic from 2002 estimated that about 3.6 percent of all cancers, or 389,100 cases, are associated with alcohol consumption (Seitz and Stickel 2007). Cancers for which strong epidemiological evidence indicates that alcohol consumption is associated with an increased risk include, but are not limited to, esophageal, laryngeal, pharyngeal, stomach, colorectal, liver, pancreas, lung, prostate, breast, central nervous system, and skin cancers (Berstad et al. 2008; Boffetta and Hashibe 2006; Brooks and Zakhari 2013; de Menezes et al. 2013; Haas et al. 2012; Kumagai et al. 2013; Longnecker et al. 1995; Nelson et al. 2013; Rota et al. 2014a; Watters et al. 2010). The risk of developing a second aerodigestive-tract cancer also is higher in alcohol drinkers (Day et al. 1994; Lin et al. 2005; Saito et al. 2014).
Increased risk of cancer often is associated with high alcohol consumption; however, the specific dose–response relationship varies according to the site of cancer. A recent meta-analysis of 16 articles involving 19 cohorts of subjects with liver cancer (i.e., hepatocellular carcinoma) found a linear relationship between the amount of alcohol consumed and the risk of liver cancer compared with nondrinkers (Turati et al. 2014). Thus, consumption of three alcoholic drinks per day was associated with a moderate increase in risk, whereas consumption of about seven drinks per day was associated with an increase in risk of up to 66 percent. A similar linear relationship has been described for breast cancer risk (Scoccianti et al. 2014).
However, alcohol consumption does not increase the risk of all types of cancer and may even be associated with a lower risk in some cases. For example, although alcohol consumption overall is associated with a higher risk of breast cancer in women, this association does not apply to all types of breast cancer. Thus, among women enrolled in the Women’s Health Initiative the risk of estrogen-positive breast cancer was increased in those who drank alcohol, whereas the risk of triple-negative breast cancer1 was reduced among drinkers compared with women who had never consumed alcohol (Kabat et al. 2011).
1 In estrogen-positive breast cancer, the cancer cells carry the estrogen receptor and depend on estrogen for growth. In contrast, in triple-negative breast cancer, the cancer cells carry neither estrogen nor progesterone or HER2 receptors.
Interestingly, alcohol consumption also is associated with a lower incidence of several types of blood cancer, including non-Hodgkin’s lymphoma (NHL) (Gapstur et al. 2012; Ji et al. 2014; Morton et al. 2005; Tramacere et al. 2012) and multiple myeloma (Andreotti et al. 2013). An analysis of 420,489 individuals diagnosed with alcohol use disorder (AUD) who were linked to the Swedish Cancer Registry also found a low risk of developing leukemia, multiple myeloma, and Hodgkin’s disease (Ji et al. 2014). Another recent study also showed that alcohol drinking was not associated with increased risk of leukemia and that, in fact, light drinking (less than or equal to one drink per day) was associated with a modest 10 percent reduction in leukemia incidence (Rota et al. 2014b). In addition to blood cancers, alcohol consumption also is associated with a lower risk of thyroid cancer (de Menezes et al. 2013) and renal cell carcinoma (Song et al. 2012). In the case of renal cell carcinoma, a lower risk was noted even with consumption as low as one drink per day in both men and women, and higher alcohol intake conferred no further benefit. Finally, a retrospective, observational study of colon and rectum adenocarcinoma indicated that moderate alcohol consumption (less than 14 grams per day) was inversely associated with the incidence of rectal cancer. The investigators also found that moderate intake of beer and especially wine was inversely associated with distal colorectal cancer (Crockett et al. 2011).
In summary, it is well established that alcohol use and abuse is associated with a wide variety of cancers, and the number of these associations continues to grow. At the same time, it now is becoming clear that alcohol can have a preventative effect for certain cancers. Whereas the role of alcohol as a carcinogen is well established, the mechanism(s) by which it prevents cancer are largely unknown and an area for further research. Also, despite the potential beneficial effects of alcohol in the prevention of some cancers, it is important to remember that the detrimental effect of chronic alcohol abuse cannot be disregarded.
Although extensive epidemiologic evidence links the etiology of cancer to alcohol, very little information addresses the critical question of whether and how alcohol modulates tumor metastasis, survival, and the response to cancer therapy. One of the components in these processes is the immune system. Much research regarding the role of the immune response in oncogenesis has centered on hepatocellular cancer (for excellent recent reviews, see Aravalli 2013; Stauffer et al. 2012; Wang 2011). However, less is known regarding the role and interaction among alcohol consumption, immune modulation of tumor growth, blood vessel formation (i.e., angiogenesis), metastasis, and survival. These issues form the major emphasis of this review. It is well established that immunosurveillance by the innate and adaptive immune systems plays important roles in the prevention of cancer and in controlling cancer survival (Fridmann et al. 2012; Rocken 2010). However, direct or indirect interactions of the tumors with their microenvironment can facilitate immune evasion so that the tumor is not detected by the immune system and thus can spread uncontrolled. Tumors also release factors that can directly or indirectly suppress antitumor immune responses, thus facilitating angiogenesis, invasion of surrounding tissues, and metastasis to distant sites in the body (for a general review, see Jung 2011). (For more information on the processes involved in tumor metastasis, see the sidebar.) The following sections will review the role of alcohol in cancer growth and progression, both in humans and in animal models.
Alcohol, Tumor Growth, and Survival in Humans
Survival and Mortality
Statistics from 2002 indicate that approximately 3.5 percent of all cancer deaths are associated with alcohol (Seitz and Stickel 2007). A study of 167,343 adult subjects in rural southern India found that daily drinking for 30 or more years increased overall cancer-related mortality (Ramadas et al. 2010). Similarly, a study involving 380,395 men and women who were followed for 12.6 years as part of the European Prospective Investigation into Cancer and nutrition (EPIC) study indicated that compared with no or light-to-moderate consumption (i.e., 0.1 to 4.9 g alcohol/day), heavy (30 or more g/day) drinking in women and heavy to extreme (60 or more g/day) drinking in men was strongly associated with increased total mortality as well as deaths from alcohol-related cancers (Ferrari et al. 2014). However, the effect of alcohol on cancer-specific mortality is variable and depends on factors such as the amount of alcohol consumed, health status of the patient, and the type of cancer.
Survival of patients with oral cavity, pharyngeal, laryngeal, and esophageal cancer is generally reduced by drinking (Jerjes et al. 2012; Mayne et al. 2009; Thrift et al. 2012; Wang et al. 2012a; Wu et al. 2012; Zaridze et al. 2009). In Korean patients with head and neck and hepatocellular carcinoma the death rate exhibited a dose-dependent relationship with consumption, with patients who drank between 124 and 289 g of alcohol per day showing the highest death rate (Park et al. 2006). Lower survival of patients with hepatocellular cancer also has been reported in Scotland (Dunbar et al. 2013), Russia (Zarizde et al. 2009), and Spain (Fenoglio et al. 2013). Shortened survival in drinkers as compared with nondrinkers with oral squamous cell carcinoma has been linked to the expression of hypoxia-inducible factor-1-alpha (HIF-1α), a biomarker associated with tumor invasion, metastasis, and progression of a variety of human cancers that also plays a central role in angiogenesis. Drinkers showed higher HIF-1α expression in the nucleus of their cancer cells than nondrinkers (Lin et al. 2008). Finally, although alcohol consumption lowers the incidence of NHL, it decreases patient survival of those with the disease (Battaglioli et al. 2006; Geyer et al. 2010; Talamini et al. 2008).
The effect of alcohol consumption on mortality of women with breast cancer is varied and difficult to interpret. In general, long-term low and moderate alcohol consumption does not seem to affect the survival of breast cancer patients (Flatt et al. 2010; Harris et al. 2012; Kwan et al. 2012; Newcomb et al. 2013). In fact, moderate drinking actually may benefit survival of young women with breast cancer (Barnett et al. 2008; Newcomb et al. 2013). On the other hand, several studies indicated that postmenopausal women with breast cancer who are high-intensity drinkers have lower survival than those with no or lower consumption (Holm et al. 2013; McDonald et al. 2002; Weaver et al. 2013).2 In addition to patient age, the specific type of breast cancer may influence the effects of alcohol on survival. Thus, for women with estrogen receptor–positive breast cancer neither pre- nor postdiagnosis alcohol consumption was associated with breast cancer mortality (Ali et al. 2014). In women with estrogen receptor–negative disease, however, mortality was slightly reduced. Another study investigated the effect of pre- and postoperative alcohol consumption over a 3-year period in 934 Swedish primary breast cancer patients who had breast cancer surgery (Simonsson et al. 2014). The study found that both pre- and postoperative consumption of any amount of alcohol was weakly associated with a lower risk of early distant metastases and death. The associations were found in patients with axillary lymph node involvement but not in patients without lymph node involvement.
2 The exception to this is a study in Russia indicating an inverse association between alcohol consumption and mortality (Zaridze et al. 2009).
The effect of alcohol consumption on the incidence as well as the mortality of patients with prostate cancer was evaluated in a prospective cohort study of 194,797 men from the United States aged 50–71 years in 1995–1996 (Watters et al. 2010). The incidence of nonadvanced prostate cancer increased with increasing number of drinks per day, with a 25 percent increase in risk observed after high alcohol consumption (six or more drinks per day). However, an inverse correlation existed between alcohol consumption and deaths from prostate cancer, suggesting that alcohol consumption likely does not affect advanced or fatal prostate cancer.
In summary, several reports indicate that alcohol consumption decreases survival of patients with cancer, whereas other studies did not observe this association. The effect of alcohol consumption on mortality of women with breast cancer is particularly complex and seems to differ according to age, estrogen receptor status, and extent of alcohol drinking. Clearly, more breast cancer–specific studies are needed that correlate mortality with the properties of the cancer and the level of alcohol consumption.
Tumor Growth and Metastasis
The actual influence of alcohol consumption on tumor growth and metastasis is largely unknown in human cancer patients. Discriminant function analysis of 39 asymptomatic Italian patients with a total of 59 small hepatocellular carcinomas arising from cirrhosis revealed that, among other variables, alcohol intake was a good predictor of tumor doubling time and 2-year survival (Barbara et al. 1992). Another study of 35 Japanese patients with hepatocellular carcinoma and type C cirrhosis found that habitual drinkers consuming 80 g of ethanol per day for 5 years had a statistically significant (P < 0.01) shorter tumor-volume doubling time than did non-alcoholic patients (78 ± 47 days vs. 142 ± 60 days) (Matsuhashi et al. 1996).
Basal cell carcinoma—a type of skin cancer—is the most common cancer in humans and continues to increase in incidence. Although the cure rate is high and mortality and morbidity rates are low, aggressive basal cell carcinomas are not rare. In a Spanish study, a significant positive association existed between moderate (5 to 10 drinks per week) and high (more than 10 drinks per week) alcohol consumption and the presence of aggressive basal cell carcinomas (Husein-Elahmed et al. 2012).
Alcohol, Tumor Growth, Invasion, and Metastasis in Animal Models
Several studies using animal cancer models indicate tumor specific differences in the effect of alcohol on tumor growth and metastasis. These models included various types of breast cancer, melanoma, lung cancer, colon cancer, and hepatocellular carcinoma (For more information, see the sidebar “Effects of Alcohol on Tumor Growth, Invasion, Metastasis, and Survival in Animal Models”). Taken together, these studies and animal models did not allow for general conclusions regarding the impact of alcohol on tumor growth, metastasis formation, and disease progression, as findings differed significantly depending on tumor type. The alcohol model used as well as the duration of alcohol administration also are important variables and can affect the overall outcome (D’Souza El-Guindy et al. 2010), as is the amount of alcohol administered. For example, in studies assessing alcohol’s effects on metastasis formation, acute administration of high doses of alcohol, which mimics binge drinking, generally increased metastasis, whereas longer-term alcohol administration either had no effect or decreased metastasis formation, depending on the amount of alcohol consumed by the animal. Several mechanisms have been suggested as to how acute alcohol may enhance metastasis formation, including alcohol-induced formation of as well as inhibition of various signaling molecules (i.e., cytokines and chemokines). However, although both of these mechanisms seem to contribute to the increase of metastases after acute administration, they do not account for the entirety of alcohol’s effects. Another mechanism whereby alcohol could facilitate metastasis of certain cancers may involve disruption of the integrity of the cells lining the blood vessels (i.e., vascular endothelium). Thus, studies found that exposure to 0.2 percent (weight per volume [w/v]) ethanol in vitro, which promotes angiogenesis and invasion, interferes with the integrity of the vascular endothelium by inducing endocytosis of VE-cadherin (Xu et al. 2012). This molecule is an important component of certain junctions between cells (i.e., cellular adherens junctions). These changes in the vascular endothelium have been shown to allow for increased migration of human A549 lung adenocarcinoma cells, MDA-MB-231 breast cancer cells, and HCT116 colon cancer cells through single-cell layers of endothelial cells (Xu et al. 2012).
Researchers also examined the effects of alcohol administration on tumor growth. These studies found that high alcohol intake had no consistent effect on tumor growth across different tumors or within a specific tumor type. Low intake of alcohol generally has been associated with enhanced angiogenesis (which promotes tumor growth), whereas high intake may have no effect.
As mentioned earlier, studies in humans found that alcohol’s effects on breast cancer, its progression, and the associated mortality are influenced at least in part by the type of breast cancer involved, specifically its estrogen receptor status. However, animal models involving different breast cancer cell lines detected no consistent trend regarding the effect of alcohol consumption on tumor growth and progression associated with estrogen receptor expression. Estrogen generally suppresses breast cancer growth in vivo but increases in vitro migration of cells away from the original tumor. However, the relationship between estrogen supplementation, diet, caloric intake, and alcohol and their effects on subcutaneous breast cancer growth seem to be highly complex.
The effects of alcohol on in vitro invasion of surrounding tissue primarily have been studied in breast cancer and melanoma cells, with a variety of results. The evidence in melanoma suggests that ethanol can positively impact the extracellular membrane and augment expression of genes that suppress tumor metastasis, resulting in inhibition of metastasis. In addition, certain immune cells called natural killer (NK) cells seem to have some role in regulating the metastasis of breast cancers and melanomas. Clearly, more mechanistic research is needed in murine models to serve as a template for further examination of the complex interactions connecting alcohol to tumor growth, metastasis, and survival in humans.
Tumor Metastasis
Tumor metastasis is the ability of tumor cells to spread from their original site to other sites in the body and to re-establish growth, a new blood supply, and tumor colonies at the new location. (1) Cells that escape from a primary solid tumor invade into the surrounding normal tissue by passing through the basement membrane and extracellular matrix (ECM). Several factors are involved in the invasion process, including the ability to activate enzymes called matrix metalloproteinases (MMP), which are important for the tumor cells to degrade basement membranes and underlying stroma. (2) The escaped cells reach the blood either directly by actively passing through endothelial cells that line the blood vessels or passively through the lymphatic system, which ultimately carries the tumor cells to the blood. (3) Once in the blood, the tumor cells exit into tissues at the secondary site from small capillaries by passing through endothelial cells and then invading the basement membrane of the ECM. (4) Once at the secondary site, the tumor cells can lay dormant for extended periods of time, or (5) they re-establish growth to form metastatic tumor colonies (by proliferation of cells from a single tumor cell), and finally form a new blood supply (by stimulating the angiogenesis process) to nourish the metastatic tumor. Dormant cells also can proliferate at a future date and ultimately establish a new metastatic tumor. Factors that control the breaking of dormancy are largely unknown, and this is an active area of research.
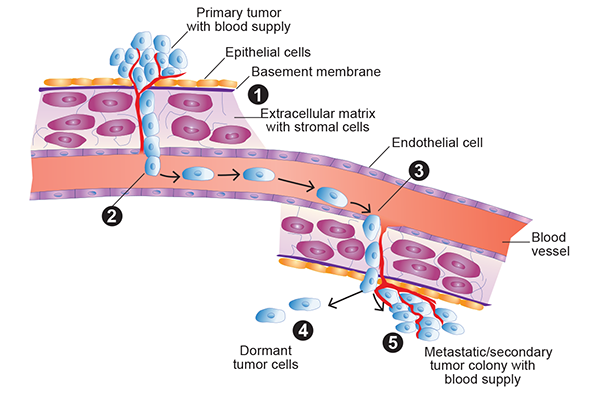
Alcohol-Induced Immune Modulation and Tumor Progression
Although many factors influence tumor growth, metastasis, and survival in cancer patients, it is apparent that a functioning immune system plays an important role, not only because it helps control cancer progression but also because it is required for the effectiveness of common cytotoxic chemotherapeutic drugs (Bracci et al. 2014). Evidence that directly implicates immune cells from both the innate and adaptive immune systems in control of cancer growth and progression continues to accumulate. This has stimulated research directed toward developing effective immunotherapeutic approaches to treat cancer (for a review of the tumor immune response as well as approaches being taken to develop immunotherapeutics for cancer, see Harris and Drake 2013).
The innate immune response reacts rapidly to recognize and destroy cancer cells. This response is characterized by inflammatory reactions involving various mediators, including chemokines and cytokines that are produced by a variety of immune cells, such as macrophages, neutrophils, NK cells, and dendritic cells. Macrophages and neutrophils can exhibit antitumor activity as well as suppress immune response against tumor cells (i.e., have immunosuppressive activity). NK cells can destroy tumors on contact, and their antitumor function can be further stimulated by cytokines. Dendritic cells are important in presenting molecules that identify a cell as harmful or foreign (i.e., antigens) to other immune cells and are a bridge between the innate immune response and the B-cell and T-cell responses that characterize the adaptive immune system.
B cells can recognize tumor-cell antigens to ultimately produce antitumor antibodies. They also can have immunosuppressive activity. T cells can be classified according to certain molecules they exhibit on their surfaces, such as CD4, CD8, or CD25. They also can be classified according to their specific functions (e.g., as helper, cytotoxic, regulatory, or memory T cells). CD4+ helper T cells can further be divided into Th1, Th2, and Th17 subpopulations based on the specific cytokines they produce and the reactions they induce in the body, which may either facilitate or suppress antitumor immune responses. Certain subsets of CD4+CD25+ T cells, known as regulatory T cells, generally are immunosuppressive. Cytokines released by Th1 helper T cells, in turn, can activate CD8+ T cells, rendering them directly cytotoxic to tumor cells as well as enhance the activity of NK cells. Other populations of CD8+ cells (i.e., tumor-specific and memory CD8+ T cells) produce high levels of the cytokine interferon gamma (IFN-γ), which is important to the control of tumor metastasis and host survival. Finally, another population of T cells (i.e., NKT cells) that produce a wide variety of cytokines upon activation can function as immunoregulatory cells to either enhance or suppress antitumor immune responses, depending on the cytokine profile that they exhibit. Together, the cells of the immune response provide an intricate interactive control that governs tumor growth and progression. (For more information on the innate and adaptive immune systems and their responses, see the “Primer on the Immune Response,” by Spiering.)
A Role for the Immune System in Control of Cancer Progression
Numerous findings with a variety of tumor types suggest that the numerous types of immune cells, particularly various T-cell subpopulations, are involved in controlling tumor progression, including the following:
- CD8+ T cells, in particular a subtype expressing the memory phenotype (CD8+CD44hi) that produce high levels of IFN-γ, are key to controlling metastasis and host survival of different tumors (Erdag et al. 2012; Eyles et al. 2010; Fridman et al. 2012; Rosenberg and Dudley 2009).
- Increased tumor progression in patients with gastric cancer has been tied to increased peripheral blood levels of certain CD4+ T-cell subpopulations, including Th22 (CD4+IL-22+IL-17-IFN-γ-) and Th17 (CD4+IL-17+IFN-γ-) cells (Liu et al. 2012).
- A multivariate analysis in metastatic breast cancer patients indicated that prolonged progression-free survival was correlated with increased CD3+CD4+ or CD8+CD28+ T cells. Conversely, elevated CD8+ CD28- T cells were associated with shortened progression-free survival (Song et al. 2013). These effects seem to be related to the cytokines produced by these cells, because patients with elevated CD8+CD28- and CD4+CD25+ T cells had elevated levels of IL-6, and the patients that expressed elevated CD8+CD28- T cells also exhibited decreased IFN-γ.
These data underscore the importance of immune cells in the progression of cancer.
Alcohol can modulate the body’s immune responses, and it is possible that these alterations affect disease progression in cancer patients. For example, in a Chinese study of newly diagnosed NHL patients (Lin et al. 2009), alcohol addiction was associated with increased peripheral blood CD4+ CD25hiCD127(IL-7)lo regulatory T cells, and these increases were higher in male than in female patients. However, the increased levels of these cells did not relate to the clinical features (e.g., age, tumor staging, cancer symptoms, pathological subtype, and short-term treatment efficacy). Therefore, the importance and significance of the elevated regulatory T cells is uncertain in NHL.
Another study of 25 patients with hepatocellular carcinoma in Japan (Yang et al. 2006) found an increase in CD4+CD25+ T cells in the tissue regions surrounding the tumor (i.e., the peritumoral region) compared with similar tissues in patients who had chronic hepatitis or liver cirrhosis but no hepatocellular carcinoma. The values were not correlated with the stage of the tumor.3 These peritumoral CD4+CD25+ T cells had a regulatory phenotype, as indicated by an increased expression of several molecules (e.g., cytotoxic T lymphocyte antigen 4 [CTLA-4, CD152] and glucocorticoid-induced TNF receptor superfamily member 18 [GITR, CD357]), expression of a biomarker for regulatory T cells (i.e., FOXP3), and decreased expression of CD45RA. The numbers of these cells were inversely associated with the numbers of CD8+ T cells. Additional observations suggest that these regulatory T cells may contribute to the progression of hepatocellular carcinoma by interfering with normal immune responses. Thus, isolated peritumor CD4+CD25+ T cells that were incubated with peripheral blood T cells from the same person and stimulated with certain antibodies, suppressed T-cell proliferation and activation of CD8+ T cells (Yang et al. 2006).
3 Interestingly, the same cell type was decreased in the peripheral blood in the cancer patients compared with control patients.
The functionality of the innate immune system also can be correlated with tumor progression. A recent study compared innate immune-system functionality with the number of circulating tumor cells in patients with a variety of cancers. In patients with metastatic disease, these circulating tumor cells are promising as biomarkers for tumor progression and overall cancer survival, with relatively high circulating cell numbers correlated with a poor prognosis. The study, which included patients with metastatic breast, colorectal, and prostate cancer found decreased NK cell cytolytic activity and decreased expression of certain proteins (i.e., toll-like receptors 2 and 4) in patients with high circulating tumor cells compared with patients with relatively low numbers (Santos et al. 2014). Decreased NK cytolytic activity also has been linked with other types of cancer, including colorectal cancer (Kim et al. 2013), metastatic melanoma (Konjevic et al. 2007), and head and neck cancer (Baskic et al. 2013).
In addition to the effects of specific types of lymphocytes on cancer growth and metastasis, chemokines also have important roles in cancer progression, terminal growth arrest of tumor cells (i.e., tumor growth senescence), angiogenesis, epithelial mesenchymal transition,4 metastasis, and evasion of the immune system. Chemokines and their receptors often are altered in cancer patients, and their importance in cancer progression has been the subject of several recent reviews (Aldinucci and Colombatti 2014; de Oliveira et al. 2014; Sarvaiya et al. 2013).
4 Epithelial mesenchymal transition is a process whereby epithelial cells lose their innate cellular polarity and cell–cell adhesive properties to become mesenchymal cells, which lack polarity and have the ability to migrate and to invade through tissues.
Alcohol and Immune Effects in Patients with Cancer
A large body of literature indicates that alcohol consumption modulates many aspects of the innate and adaptive immune systems. Alcohol originally was described as immunosuppressive, and numerous studies support the immunosuppressive aspects of alcohol consumption on the innate and adaptive immune systems. However, it also is well documented that chronic alcohol administration can activate the immune system—especially dendritic cells, T cells, and NKT cells—in experimental animals as well as humans (Cook et al. 1991; Laso et al. 2007; Song et al. 2002; Zhang and Meadows 2005). This adds to the complexity of interpreting alcohol’s effect on cancer progression and survival.
Few studies have specifically examined the interaction between alcohol and the immune response in cancer patients or in experimental animals implanted with cancer cells. Although human cancer patients often have immune deficits, few data are available that specifically address the effects of alcohol on immune parameters. The studies that are available examined the immune responses in patients with head and neck cancer. These patients often are immunodeficient because of their alcohol abuse and heavy tobacco use; however, the contribution of continued alcohol abuse to altered immune parameters in these patients has largely not been assessed.
An early study of patients with head and neck squamous cell carcinoma and a history of smoking and significant alcohol use found a deficiency in the percentage of certain T cells (i.e., Th5.2+ IL-2–producing T cells) in peripheral blood compared with control patients who were hospitalized for elective surgical procedures (Dawson et al. 1985). The overall percentage of all T cells, as well as of CD4+ T-, CD8+ T-, B-, and NK cells, in contrast, did not differ between cancer and control patients. However, this effect cannot be clearly attributed to alcohol because the patients also were heavy tobacco users. Another study compared a different indicator of immune-system function (i.e., production of antigen-specific antibodies) using blood samples obtained from patients with squamous cell carcinoma of the oropharynx or larynx and healthy controls, some of whom had high alcohol consumption (i.e., 100 g/day) and/or excessive smoking (20 cigarettes per day for more than 5 years) (Wustrow 1991). The study found that among healthy participants, those with high alcohol consumption or smoking had a pronounced decrease of antigen-specific antibody production in vitro. The effect was more pronounced in heavy drinkers than in excessive cigarette smokers. Cancer patients who were heavy drinkers, in contrast, did not show any antigen-specific antibody production in vitro. However, after removal of a subset of white blood cells (i.e., mononuclear cells) from the peripheral blood, samples from two-thirds of the patients began to produce such antibodies, and antibody production reached the same level as that measured in the healthy subjects with high alcohol abuse and cigarette consumption. The author suggested that the decreased antigen-specific antibody production in the cancer patients could be related to upregulation of suppressive cells in these patients (Wustrow 1991).
More recent studies have evaluated the role of a protein called macrophage migration inhibitory factor (MIF), which is an important regulator of the innate immune response. This factor has been studied in patients with lip or intra-oral squamous carcinoma as well as in patients who consumed alcohol regularly (Franca et al. 2013). The analyses found a significant relationship between the incidence of intra-oral cancer, alcohol use, and the number of MIF-positive cells in the stroma. Thus, MIF in the stroma of intra-oral tumors (i.e., tongue, floor of mouth, and alveolar ridge) was decreased in patients who consumed alcohol. The importance of these findings is unknown, although patients with tumors that did not express MIF had a worse prognosis than patients that did.
Alcohol and Immune Interactions in Animal Models of Cancer
If human tumor cells are introduced (i.e., inoculated) into animals with functioning immune systems, they do not form tumors because they are recognized as foreign by the animal’s immune system. However, human tumors often grow in animals with compromised immune systems, and such animals can be used as models for a variety of research questions, including studies regarding the roles of various immune cells in controlling cancer and the impact of alcohol on this process. One such study specifically examined the role of CD4+ T cells in regulating tumor growth by implanting cells from a human lung cancer (i.e., the 201T human lung adenocarcinoma cell line) into the lungs of a strain of mice called BALB/c (Hunt et al. 2000). In this study, the mice were administered alcohol chronically for 8 weeks and then were injected with an anti-CD4 monoclonal antibody to deplete CD4+ T cells. Initial experiments confirmed that normal, immunocompetent BALB/c mice did not form lung tumors. To examine the effect of alcohol, the mice were administered ethanol in their food5 as well as 10 percent in their drinking water throughout the experimental period. After 8 weeks of ethanol administration or regular food, the mice were implanted with the tumor cells and also received one injection of the anti-CD4 antibody. Separate groups of mice were evaluated at 6 weeks and 13 weeks. Mice in the non–ethanol-fed control group injected with one dose of anti-CD4 antibody initially developed large tumors at 6 weeks, which significantly regressed thereafter. Compared with these control animals, the ethanol-fed mice exhibited significantly larger tumors at 6 weeks as well as a diminished ability to decrease their tumor size at 13 weeks. The findings suggest that this difference in the ability of the ethanol-fed mice to reduce their tumor burden results from an impaired immune system caused by chronic alcohol intake.
5 The food included blocks of a jelly-like material (i.e., agar-agar) containing 40 percent alcohol and 0.5 g/kg peanut butter.
Another series of studies analyzed the interaction between chronic alcohol consumption and immune-system functioning in female C57BL/6 mice implanted with B16BL6 melanoma cells under the skin (i.e., subcutaneously). In these studies, the animals continuously received 20 percent w/v ethanol in the drinking water and generally were inoculated with B16BL6 melanoma after 12 weeks or longer of this treatment. The analyses found that in the alcohol-exposed, melanoma-bearing animals the overall numbers of peripheral blood lymphocytes (which include various types of immune cells) were lower than in water-drinking controls when determined 11, 14, and 17 days after tumor inoculation (Zhang et al. 2012). This was in contrast to normal mice not injected with melanoma cells, in which the number of lymphocytes was not altered by alcohol. The decrease in cells was not caused by cell death (i.e., apoptosis). Additional analyses demonstrated that the lowered lymphocyte numbers (i.e., lymphopenia) were associated with a two- to fourfold decrease in mature B cells as well as in CD4+ and CD8+ T cells. Further examination demonstrated that the decrease in mature B cells in the blood was associated with impaired B-cell circulation resulting from a down regulation in the formation of compound called sphingosine-1-phosphate and its receptors. Formation of sphingosine-1-phosphate is mediated (i.e., catalyzed) by an enzyme called sphingosine kinase 1, which is an important regulator of tumor progression in melanoma and several other cancers (Meng et al. 2014). This enzyme and other components of the sphingosine-1-phosphate pathway currently are being examined as potential targets for cancer drug development (Pyne and Pyne 2013; Tabasinezhad et al. 2013). Zhang and colleagues (2012) concluded that the severe decrease in mature B cells in the blood of the alcohol-exposed and tumor-inoculated animals could result from inhibition of B-cell migration from the spleen to the blood resulting from impairment of the sphingosine-1-phosphate signaling pathway. The importance and role of mature B cells in antitumor immune responses is still unclear. They play a dual role by both inhibiting (Inoue et al. 2006) and facilitating antitumor immune response through production of cytokines and enhancement of T-cell activation (DiLillo et al. 2010). Thus, impaired circulation of B cells attributed to alcohol consumption (Zhang et al. 2012) could negatively affect T-cell function.
The investigators also analyzed the levels of the various types of blood cells in the spleen (Zhang et al. 2012). The spleen contains proportionally more B cells and fewer T cells than the peripheral blood; among the T cells, the spleen normally contains a higher proportion of CD8+ T cells than the peripheral blood. The analyses found that alcohol consumption also led to a decrease in CD8+ T cells in the spleen; however, this reduction was less remarkable than in peripheral blood. No changes in these cells were observed in the bone marrow. Furthermore, alcohol consumption reduced the overall numbers of B cells in the spleen, although it did not affect all types of B cells equally. Thus, there was no effect on splenic follicular B cells, whereas the number of immature T1 B (CD19+CD93+CD23-) cells increased and the number of marginal zone B cells (CD19+CD1dhiCD21hi) decreased.
Other analyses (Zhang and Meadows 2010) investigated the effects of chronic alcohol consumption on various types of CD8+ T cells in mice with or without inoculation of B16BL6 melanoma cells. These analyses yielded the following results:
- CD8+CD44hi T memory cells produced high levels of IFN-γ and were important in the antitumor response to B16BL6 melanoma. Mice not inoculated with melanoma that chronically consumed alcohol had higher levels of these memory cells than mice that drank water.
- After melanoma inoculation, these CD8+CD44hi T memory cells increased over a 2-week period in water-drinking animals. However, in mice that chronically consumed alcohol, these memory cells failed to expand in response to melanoma inoculation.
- The lack of expansion of the memory T cells in response to melanoma inoculation in the alcohol-consuming mice resulted from a reduced ability of these cells to proliferate in response to melanoma. Additional experiments examined the ability of CD8+ T cells obtained from 2-week melanoma-bearing mice to proliferate in vitro in response to specific T cell stimulation (i.e., anti-CD3 and anti-CD28 anti- bodies). The analyses showed that proliferation of CD8+ T cells was reduced by more than one-half in alcohol-consuming mice compared with cells from water-drinking mice.
- The number of CD8+ T cells that specifically recognize a melanoma-specific antigen (i.e., gp100) was 2.5-fold lower in the spleen of the alcohol-consuming mice than in water-drinking control mice at three weeks after tumor inoculation, suggesting an impaired immune response.
- The percentage of IFN-γ–producing CD8+ T cells, which have tumor-suppressive effects, initially displayed a robust increase until day 11 after melanoma inoculation, but exhibited an accelerated decay thereafter, suggesting enhanced inhibition of these cells related to an alcohol–melanoma interaction.
The investigators also analyzed the numbers of several types of cells whose production is induced by tumors and which produce factors that inhibit the antitumor functions of T cells, including myeloid-derived suppressor cells (MDSCs), tumor-associated macrophages, T regulatory cells (CD4+CD 25+FOXP3+), regulatory B cells (CD1dhiCD5+), and NKT cells (Zhang and Meadows 2010; Zhang et al. 2012). Of these, the percentage of CD11b+Gr-1int MDSCs, as well as the percentage of the CD124+ subpopulation within the CD11b+Gr-1int cells, was increased in the peripheral blood of alcohol-consuming mice as determined one week after tumor inoculation. These cells are known to suppress antitumor T-cell immune responses (Sinha et al. 2005; Terabe et al. 2005; Zhu et al. 2007). The percentages of T regulatory cells and tumor-associated macrophages did not differ between alcohol-consuming and water-drinking mice with melanoma tumors (Zhang and Meadows 2010).
The percentage and number of CD3+NK1.1+ invariant NKT cells was elevated in the blood of alcohol- consuming, B16BL6 melanoma-bearing mice especially at day 14 after tumor inoculation (Zhang et al. 2012). These cells have important regulatory functions and can either promote antitumor immune responses or inhibit them. Initially, these cells express a cytokine profile that favors antitumor immune responses (i.e., a high ratio of IFN-γ to IL-4). After repeated activation, however, these cells become anergic and switch to a cytokine profile that inhibits antitumor immune responses and favors tumor progression (i.e., a high ratio of IL-4 to IFN-γ) (Parekh et al. 2005). The invariant NKT cells from the alcohol-consuming, melanoma-bearing mice exhibit a high IL4/IFN-γ ratio, indicating that they express a cytokine profile favoring immune inhibition and tumor progression (Zhang et al. 2015).
Overall, very few studies have addressed the role of and interaction among alcohol, cancer, and the immune system once the cancer is established. It is important to understand these interactions, however, because many alcoholics have immune deficiencies and because a competent immune system is important to the success of many conventional drug therapies for cancer. In addition, new immune- enhancing approaches to cancer therapy are being developed. Finally, evidence from animal models and human studies suggests that appropriately combined chemotherapy and immunotherapy may be more beneficial than either therapeutic approach alone (Ardiani et al. 2013; Shi et al. 2014; van Meir et al. 2014; Wang et al. 2014).
Glossary
Antibody: Immune molecule (protein) produced by B cells that recognizes foreign molecules that have entered the body (i.e., antigens), binds to these molecules, and marks them for destruction by the body’s immune system.
Antigen: Any molecule that can bind specifically to an antibody and can induce an immune response.
B cells: One of the two main types of lymphocytes involved in the adaptive immune response; when activated by interacting with a specific antigen, they differentiate into specific subtypes and begin to produce antibodies that recognize the specific antigen.
Chemokines: Small proteins that serve as chemoattractants, stimulating the migration and activation of cells, particularly phagocytic cells and lymphocytes; they have a central role in inflammatory responses.
Cytokine: Any of a group of molecules, produced primarily by immune cells, that regulate cellular interactions and other functions; many cytokines play important roles in initiating and regulating inflammatory reactions.
Dendritic cell: A type of immune cell involved in the innate immune response that is characterized by a branched morphology; dendritic cells can bind to antigens and present these antigens to T cells, thereby initiating an adaptive immune response.
Macrophage: A type of immune cell that ingests foreign particles and micro-organisms in a process called phagocytosis and which synthesizes cytokines and other molecules involved in inflammatory reactions.
Natural killer (NK) cell: A type of immune cell involved in the innate immune response that can kill certain harmful cells, particularly tumor cells, and contributes to the innate immune response to cells infected with viruses or other intracellular pathogens.
Neutrophil: A type of immune cell involved in the innate immune response that engulfs and kills extracellular pathogens in a process called phagocytosis.
T cells: One of the two main types of lymphocytes involved in the adaptive immune response after activation through the interaction with a specific antigen. T cells can be divided into several subgroups that support other immune cells (helper T cells), kill invading pathogens or infected cells (cytotoxic T cells), or help turn off the adaptive immune response (regulatory
Additional Avenues for Future Research
The interactions between alcohol use/abuse, the antitumor immune response, tumor growth, and spread of cancer are complex. A negative impact of alcohol on the immune system can lead to increased cancer mortality; however, studies also indicate that alcohol, generally in low doses, can have beneficial effects on mortality, depending on the cancer. Clearly, more mechanistic research is needed to define the complex interactions between cancer and alcohol. Additional research is likely to uncover targets to mitigate the detrimental effects of alcohol on mortality and to identify specific biochemical and molecular mechanisms involved in the beneficial effects of alcohol related to enhancing survival of cancer patients. This research could translate into the development of more effective and specific targeted approaches to treat cancer patients in general and especially those who abuse alcohol.
Because cancer is a collection of different diseases with diverse underlying causes, it is important that research take into account the diversity in gene mutations and alterations involved in uncontrolled growth. In addition, future analyses must address the genetic instability that fosters metastasis, the major cause of death from cancer. It is becoming increasingly clear that genes which suppress metastasis (Meadows 2012) as well as signaling pathways that inhibit metastasis (Singh et al. 2014) can be regulated through epigenetic mechanisms6 induced by the diet and dietary constituents, including alcohol. Alcohol-related epigenetic mechanisms include modulation of DNA methylation, histone acetylation/deacetylation, and expression of micro RNA (French 2013). These epigenetic mechanisms associated with alcohol also are known to affect the gastrointestinal-hepatic system (Shukla and Lim 2013) and may promote, for example, the progression of hepatic carcinoma. For the most part, alcohol-related epigenetic changes have not yet been associated with tumor growth, metastasis, and survival; however, alcohol-induced aberrant DNA methylation of certain genes plays a role in the control of breast cancer (Tao et al. 2011). Moreover, alcohol also can dysregulate the immune system through epigenetic mechanisms (Curtis et al. 2013), and this aspect of the association between alcohol, the immune system, and cancer progression needs to be explored further.
6Epigenetic mechanisms are processes that alter the expression of certain genes without permanently altering the DNA building blocks (i.e., nucleotides) making up the genes; examples of epigenetic mechanisms include the temporary chemical modification (e.g., methylation or acetylation) of nucleotides or of the proteins (i.e., histones) around which the DNA is wrapped in the cell nucleus.
Another potential target for future research is a molecule called toll-like receptor 4, which is known to help regulate host innate immunity. This receptor recognizes the lipopolysaccharide (LPS) endotoxin, a molecule found on by certain bacteria that are part of the intestinal microflora. In the blood, LPS can induce strong immune reactions. Alcohol is known to facilitate the release of LPS from the gut into the systemic circulation, and this is a key factor in the pathogenesis of alcoholic liver disease (Petrasek et al. 2010). In addition to its response to LPS, toll-like receptor 4 can facilitate antitumor immune responses; however, emerging evidence also suggests that overactivation of this receptor is associated with tumor progression as well as tumor development (Mai et al. 2013). Although these observations need to be explored further, they suggest that this receptor could be a target for future agonist or antagonist targeted treatment for cancer, particularly for patients that abuse alcohol.
Continued research into the detrimental and beneficial effects of alcohol in human cancer patients and animal models of cancer is a key factor to understanding the complex interactions that affect tumor progression and survival, particularly in the context of alcohol use. This research has a strong potential to discover new immunotherapy and epigenetic approaches to cancer treatment as well as treatment of other alcohol-induced diseases.
Effects of Alcohol on Tumor Growth, Invasion, Metastasis, and Survival in Animal Models
Several studies using animal cancer models indicate tumor-specific differences in the effect of alcohol on tumor growth and metastasis. These models included various types of breast cancer, melanoma, lung cancer, colon cancer, and liver cancer (i.e., hepatocellular carcinoma).
Lung Cancer
One early study (Capel et al. 1978) investigated the effect of alcohol exposure on the growth and metastasis of Lewis lung carcinoma. Male animals from a type of mouse strain called C57BL/6 were exposed to 10 percent ethanol in their drinking water for 2, 4, 5, or 8 weeks before tumor cells were implanted into their thighs. Ethanol administration then was continued for 2 more weeks. The study found that ethanol exposure before tumor injection did not affect tumor growth. Furthermore, metastases were significantly reduced in the 2-week and 8-week ethanol groups but not in the 4-week and 5-week groups. Administration of ethanol for 2 weeks after tumor inoculation affected neither tumor growth nor metastasis. These investigators also evaluated the effect of 2-week pre- and postimplantation ethanol exposure (10 percent in drinking water) on the growth of another type of tumor called Ehrlich ascites carcinoma, which is a spontaneous murine mammary adenocarcinoma adapted to grow in fluid in the abdominal cavity (i.e., ascites). Exposure to ethanol before but not after tumor injection significantly decreased the tumor cell number.
Colon Cancer
Gu and colleagues (2005) assessed the effects of alcohol on human HT1080 colon cancer cells in a chick embryo model, focusing on variables related to the blood supply of the tumor. One of the variables analyzed was the expression of vascular endothelial growth factor (VEGF)—a growth factor that promotes blood vessel formation (i.e., is proangiogenic) and enhances tumor vascularization. The study found that exposure to 0.25 g/kg ethanol per day for 9 days resulted in a 2.2-fold increase in tumor volume as well as a 3.9-fold increase in the expression of VEGF mRNA in the tumor cells and a 2.1-fold increase in the amount of the blood vessels in the tumor (i.e., intratumoral vascular volume) compared with control embryos. Exposure of isolated tumor cells to 10 mM and 20 mM ethanol for 19 hours also increased VEGF mRNA and protein expression. The increased intratumoral vascular volume strongly correlated with the increase in tumor volume as well as with intratumoral connective tissue volume density. Finally, invasion of HT1080 cells from the tumor into blood vessels (i.e., intravasation), which occurs during metastasis, increased more than eightfold in response to ethanol. Ethanol did not have a direct effect on cell proliferation.
Hepatocellular Carcinoma
Because excessive alcohol consumption often is associated with liver disease, investigators also have examined the effects of alcohol on hepatocellular carcinoma. One study (Thompson et al. 2013) evaluated the effects of alcohol as well as a high-fat diet on hepatocellular carcinoma progression in male C57BL/6 mice with diet-induced obesity as well as in nonobese control mice. Both groups were injected with Hepa1-6 tumor cells. Ethanol was administered in drinking water at daily alternating concentrations of 10 percent and 20 percent for 6 weeks before the animals were injected with the tumor cells. The alcohol and dietary regimens then were continued for an additional 8 weeks. Gross numbers of tumors as well as tumor burden were lower in mice fed the high-fat diet with and without ethanol or the control diet with ethanol than in control animals receiving neither ethanol nor high-fat diet, with ethanol exerting a greater protective effect than the high-fat diet. However, the high-fat diet both alone and in conjunction with ethanol enhanced mortality in the tumor-bearing mice. Further analyses found that all tumor-bearing mice exhibited increased numbers of inflammatory white blood cells in their livers, regardless of whether they had received ethanol. The expression of some cytokines and their receptors also was altered in the tumor-bearing mice, and these changes in some cases depended on the animals’ diet. Thus, tumor necrosis factor (TNF)-α and interleukin (IL)-6 receptor alpha mRNA expression were increased and transforming growth factor (TGF)-b mRNA expression was decreased in tumor-bearing mice receiving the high-fat diet compared with mice not injected with tumor. An analysis of seven cytokines (IL-1b, IL-6, IL-10, IL-12, IL-13, interferon gamma [IFN-γ], and TNF-α) in the pooled plasmas from each experimental group indicated no changes across the groups except in the water-drinking, high-fat diet group, which exhibited depressed cytokine levels. There was no correlation with ethanol consumption compared with mice not injected with tumor cells.
Breast Cancer
The effect of ethanol on mammary cancer growth has been studied in a number of animal models, using both rodent and human tumor cell lines.
Studies Using Rodent Tumor Cell Lines
Wang and colleagues (2012) examined the effect of ethanol on the growth of the aggressive estrogen receptor–positive E0771 mouse mammary cancer in female C57BL/6 mice. The mice were given 2 percent ethanol in drinking water for half a day on each of 3 consecutive days before the E0771 tumor cells were inoculated into breast tissue (i.e., secondary mammary fat pad), and the ethanol feeding regimen then was continued for 24 days. The study found that the ethanol group exhibited higher primary tumor growth rates, increased final tumor weights, and a twofold increase in lung metastases compared with the water-drinking control group. Immunohisto-chemical analyses of the mammary tumor tissues also showed a higher density of tiny blood vessels in the ethanol group, indicating that ethanol promoted tumor angiogenesis. Finally, the investigators found increased expression of a chemokine called monocyte chemoattractant protein-1, or MCP-1 (also known as CCL2), which has been implicated in tumor development and angiogenesis, in tumor tissues from the ethanol group and in E0771 cells exposed to 0.2 percent ethanol. MCP-1 plays an important role in suppressing antitumor immune functions and facilitating tumor metastasis (Kudo-Saito et al. 2013), indicating another mechanism through which alcohol could promote breast cancer progression.
A related study using the same alcohol-feeding regimen confirmed alcohol’s effects on growth and angiogenesis of E0771 inoculated into other female C57BL/6 mice (Lu et al. 2014). In that study, a molecule that can inhibit VEGF receptor 2 blocked alcohol’s stimulatory effect on tumor growth, indicating that alcohol acts via a VEGF pathway.
Holcomb and colleagues (2012) examined the effects of various diets and supplements on the growth of estrogen receptor–positive mammary tumor cells (derived from mammary tumor virus-Wnt-1 transgenic mice) inoculated subcutaneously into female C57BL/6J mice that had their ovaries removed. Conditions tested included (1) 20 percent weight per volume (w/v) alcohol in drinking water; (2) a calorie-restricted diet with 30 percent fewer calories than normal; (3) a high-fat diet where 60 percent of calories were derived from fat; (4) a low-fat diet where 10 percent of calories were derived from fat; and (5) estrogen supplementation. The diets and alcohol were started when the animals were 8 weeks old and continued for 27 weeks. Estrogen pellets were implanted after 19 weeks of alcohol consumption, and tumors were implanted after 22 weeks. The results on tumor growth were similar to those obtained by Hong and colleagues (2011), with the high-fat diet and alcohol promoting tumor growth and estrogen suppressing it. Tumor growth was greatly inhibited in the mice receiving a high-fat diet as well as estrogen supplements. The calorie-restricted diet also inhibited tumor growth independent of the effects of alcohol and estrogen supplementation. Neither the diets nor alcohol affected VEGF.
Researchers also studied the effects of alcohol on estrogen receptor–negative mouse mammary tumors. One study involving estrogen receptor–negative Met-1 cancer cells used female FVB/N mice that consumed 20 percent w/v ethanol in drinking water for 18 weeks before they were injected subcutaneously with the cancer cells (Hong et al. 2010). Compared with water-drinking control mice, the ethanol-drinking animals developed palpable tumors earlier and also developed larger tumors. Several other parameters (i.e., insulin sensitivity, leptin levels in the blood, and estrogen levels) were elevated in the alcohol-consuming mice. These researchers also examined the effect of ethanol in vitro on the migration of the estrogen receptor–positive T47D breast cancer cell line. The results showed that cells exposed to different concentrations of ethanol from 0.1 percent to 0.5 percent exhibited increased migration, as did cells exposed to estrogen (20 nM). The combination of estrogen and 0.5 percent resulted in higher migration than either treatment alone.
In another study (Hong et al. 2011), the researchers examined the effects of alcohol on Met-1 tumor growth as a function of a diet (i.e., low fat vs. high fat) and estrogen supplementation (low dose vs. high dose). Mice consuming the high-fat diet developed larger tumors than did mice fed the low-fat diet; moreover, alcohol ingestion increased the final tumor volume in both dietary groups. Estrogen treatment suppressed tumor growth regardless of diet and alcohol consumption; however, mice treated with high-dose estrogen had slightly larger tumors than did mice treated with the low dose. Tumor tissues also were analyzed for the levels of various regulatory molecules hypothesized to potentially influence tumor progression, including phosphoinositide 3 kinase (PI3K/p85α); a protein kinase called Akt; the phosphorylated form of Akt (p-AktSer473), a signaling molecule associated with cell proliferation, survival, and invasion; proliferating cell nuclear antigen (PCNA); and cleaved caspase-3, a molecule involved in programmed cell death (i.e., apoptosis), with the following results:
- PI3K (p85α) was not affected by alcohol, high-fat diet, or estrogen treatment.
- Alcohol and the high-fat diet increased expression of p-AktSer473. In contrast, estrogen supplementation reduced phosphorylation of Akt in both the alcohol and high-fat diet groups.
- The high-fat diet and alcohol decreased the level of cleaved caspase-3, suggesting decreased apoptosis in the tumor tissue. Interestingly, estrogen reduced this signaling molecule in tumors from mice fed alcohol and high-fat diets.
- PCNA was not altered by any of the variables, indicating that cell proliferation was not affected.
Other researchers investigated the effects of alcohol on metastasis of the estrogen receptor–negative and natural killer (NK) cell-sensitive rat MADB106 mammary adenocarcinoma (Yirmiya et al. 1992). In this study, male Fischer 344 rats were administered only one alcohol dose (1.5 to 3.5 g ethanol/kg body weight into the peritoneal cavity) 1 hour before intravenous tumor inoculation. The higher ethanol doses (i.e., 2.5 g/kg and 3.5 g/kg) significantly increased the number of lung metastases, whereas the lowest dose (1.5 g/kg) did not. Administration of naltrexone, an opioid receptor antagonist used to treat alcohol dependence, did not modify the alcohol-related increase in metastasis. Ethanol’s effects on lung metastasis seem to depend on the exact administration schedule. Thus, in a related study these researchers found that administration of 2.5 g/kg ethanol 24 hours before or after tumor inoculation did not affect lung metastasis (Ben-Eliyahu et al. 1996). Yirmiya and colleagues (1992) also administered ethanol in a liquid diet for 2 weeks before and 3 weeks after tumor inoculation and found that lung metastases were increased.
Alcohol’s effects also seem to depend on the tumor type studied. When rats were injected intravenously with rat CRNK-16 leukemia cells instead of MADB106 cells, acute administration of 1.5 to 3.5 g of ethanol/kg/body weight reduced survival in a dose-related fashion, whereas maintenance on a liquid diet containing 5 percent w/v ethanol did not (Ben-Eliyahu et al. 1996; Yirmiya et al. 1992).
One of the ways in which the body defends itself against tumor cells involves their destruction by NK cells. The investigators also analyzed alcohol’s effects on NK-cell activity, finding that neither acute injection nor dietary administration of ethanol in these experiments affected NK-cell activity against MADB106 cells when determined in an in vitro assay (Yirmiya et al. 1992). When MADB106 and CRNK-16 cells were incubated with ethanol in vitro, the numbers of these cells were reduced after 5 days. These effects were significant for MADB106 cells at ethanol concentrations of 0.2 percent, 0.5 percent, and 1.0 percent ethanol and for CRNK-16 cells at 0.5 percent and 1.0 percent ethanol. Ethanol had no effect in rats that were depleted of NK cells, and metastasis was not affected after injection of the NK-insensitive C4047 rat colon cancer cell line (Ben-Eliyahu et al. 1996).
Tumor growth and metastasis also were studied in the highly metastatic estrogen receptor–negative 4T1.2 cells implanted into the mammary fat pad of female Balb/c that had consumed alcohol in drinking water for 4 weeks (Vorderstrasse et al. 2012). The analyses indicated that continuous availability of 1 percent, 5 percent, and 18 percent ethanol w/v did not affect either the establishment of tumors or the final mammary tumor weight, indicating a lack of effect on tumor growth. However, the animals receiving 18 percent ethanol exhibited a marked reduction in metastasis to secondary mammary glands and to the lung. Tumor-associated increases in spleen size (i.e., splenomegaly) also were significantly reduced in the 18-percent ethanol group. Lung metastasis tended to be lower in mice consuming 5 percent ethanol, but there was no effect among those consuming 1 percent ethanol. Although the animals receiving 18 percent ethanol showed suppressed metastasis, they were less healthy compared with the other treatment and control groups as indicated by appearance, body condition, natural behavior, and provoked behavior. In vitro studies indicated that exposure of cells to 0.3 percent w/v ethanol did not affect 4T1.2 cell proliferation, colony formation, or invasion but did reduce cell migration twofold. Reduced cell migration may be related to changes in expression of CXCR4, a chemokine receptor that is important to migration and metastasis of breast and other cancers (Sarvaiya et al. 2013). Vorderstrasse and colleagues (2012) showed that expression of CXCR4 was suppressed 60 to 80 percent in tumors of mice consuming 18 percent ethanol; however, a correlation analysis indicated a lack of relationship between CXCR4 expression and metastasis. When the 4T1.2 cells were grown in 0.3 percent ethanol, however, CXCR4 expression was not altered, suggesting that expression was indirectly modulated by alcohol consumption in vivo.
Studies Using Human Tumor Cell Lines
Several studies examined the specific effects of ethanol on various aspects of disease progression in human breast cancer cell lines, including proliferation of cells. Singletary and colleagues (2001) found that incubation in 0.4 percent w/v ethanol increased cell proliferation in the estrogen receptor– positive MCF-7 and ZR75.1 breast cancer cells but not in the estrogen receptor– negative BT-20 and MDA-MB-231 cells. The effect of ethanol on MCF-7 cells also was correlated with increases in estrogen receptor alpha content. However, alcohol’s effects are complex. When the MCF-7 cells were cultured together with human skin fibroblasts in 0.4 percent ethanol for 72 hours, ethanol suppressed estrogen receptor alpha expression compared with untreated cells (Sanchez-Alvarez et al. 2013). Thus, the tumor micro-environment is important in determining estrogen-receptor status and the effects of alcohol on breast cancer. Whether this study is relevant to patients with breast cancer is not known. However, additional studies are warranted, because estrogen receptor–negative breast cancer generally is more aggressive, and patients have a worse prognosis than patients with estrogen receptor–positive breast cancer. Moreover, conversion from estrogen receptor alpha positive to estrogen receptor alpha negative can occur (Hoefnagel et al. 2010).
Other studies focused more on the invasion and migration in vitro of estrogen receptor–positive and estrogen receptor–negative human breast cancer cells. One study (Ma et al. 2003) compared the effects of incubation in 0.4 percent w/v ethanol for 48 hours on various breast cancer cell lines. This treatment increased invasion of the estrogen receptor–positive MCF-7 and T47D breast cancer cells as well as the estrogen receptor–negative HS578T, MDA- MB231, and MDA-MB435 cells. Similarly, incubation for 48 hours in 0.1 percent and 0.2 percent w/v ethanol stimulated invasion of estrogen receptor–negative SKBR3 and estrogen receptor–positive BT474 breast cancer cells. In contrast, ethanol exposure did not affect invasion of HB2, an immortalized normal human breast tissue cell line, or estrogen receptor–negative BT20 breast cancer cells. The effects of ethanol may depend not only on the specific cell line examined but also on the ethanol concentration used. Thus, studies from another laboratory demonstrated that exposure to 0.1 percent, 0.2 percent, and 0.5 percent w/v ethanol enhanced invasion of T47D, MCF-7, and MDA-MB231 cells in a dose- dependent manner (Wong et al. 2011). Similarly, Aye and colleagues (2004) examined the effects of exposure for 48 hours to different ethanol concentrations on estrogen receptor– negative SKBR3 and estrogen receptor–positive BT474 breast cancer cells. For both SKBR3 and BT474 cells exposure to 0.1 percent and 0.2 percent w/v ethanol stimulated invasion. A higher dose of 0.4 percent w/v ethanol, however, inhibited invasion of SKBR3 cells and created mixed results for BT474, with one study (Aye et al. 2004) detecting no effect on invasion and another study (Xu et al. 2010) detecting increased invasion.
Invasive ability generally was related to the expression of ErbB2/neu, an epidermal growth factor (EGF) receptor that is amplified in 20 to 30 percent of breast cancer patients, with higher ErbB2/neu levels indicating higher risk of lymph node metastasis and poor prognosis. More detailed studies of the relationship between alcohol, ErbB2/neu, and invasion in the human breast cancer cell line T47D found that activation of the EGF receptor by addition of EGF did not significantly affect ethanol’s ability to enhance invasiveness (Luo and Miller 2000). Conversely, prevention of ErbB2/neu production inhibited the ability of ethanol to increase migration (Luo and Miller 2000).
Numerous studies have sought to identify the mechanisms through which ethanol affects growth and migration of breast cancer cells or the molecules that mediate these effects. The results of such investigations include the following:
- Studies in MDA-MD231 and T47D cells determined that ethanol induced altered adhesion to fibronectin, increased development of lamellipodia,1 increased phosphorylation of a protein called focal adhesion kinase, and increased production of several proteins (e.g., ribosomal protein L7a, smooth muscle myosin alkali light chain), all of which can promote cell migration (Xu et al. 2010; Zhu et al. 2001).
- Exposure of T47D cells to 0.5 percent w/v ethanol decreased mRNA expression of NM23, a known metastasis suppressor gene (Wong et al. 2011). However, gene expression levels of two other metastasis suppressors, KISS1 and MKK4, were increased by ethanol. Ethanol treatment also modulated the expression of more than 80 other genes associated with regulation of the extracellular matrix and cell adhesion. For example, ethanol induced expression of the gene encoding fibronectin receptor subunit integrin alpha 5 (ITGA5). This receptor activates signaling pathways supporting invasion, and reduced expression of ITGA5 significantly inhibits invasion. The ethanol-induced expression of this gene was blocked by overexpression of the NM23 gene, indicating an important relationship between these two genes in controlling invasion of T47D breast cancer cells.
- Studies also assessed alcohol’s interactions with enzymes called matrix metalloproteinases (MMPs) such as MMP-2 and MMP-9 that are involved in the breakdown of the extracellular matrix in normal physiological processes as well as in disease processes, such as metastasis. Studies to date indicate no interactions between alcohol and MMPs (Aye et al. 2004; Ranuncolo et al. 2002).
1Lamellipodia are projections on the mobile edge of a cell that allow the cell to move across a substrate.
Melanoma
In one of the first experiments conducted in melanoma, 6- to 8-week-old female CDBA/2F1 mice consumed water or 20 percent alcohol for 52 weeks before being inoculated in a leg with the Cloudman 8-91 melanoma tumor (Ketcham et al. 1963). When the tumors reached a size of 1.5 to 2.0 cm (about 28 days after tumor inoculation), the groups were divided in half, and half of each group had the primary tumor- bearing leg amputated. At 56 days after tumor implantation, the number and size of pulmonary metastases were recorded for all animals. The study detected no substantial or consistent effect of alcohol on the size or incidence of pulmonary metastases. However, surgical removal of the tumor-bearing leg decreased pulmonary metastasis in both ethanol-drinking and water-drinking groups.
Other studies in mice assessed the effects of acute and chronic alcohol consumption on tumor growth and metastasis using B16 melanoma and its more metastatic variants, B16F10 and B16BL6. An early study (Capel et al. 1978) found that mice given 10 percent ethanol in drinking water for 2 weeks before inoculation with B16 melanoma into the thigh showed no altered tumor growth or metastasis compared with water-drinking controls. In another study (Tan et al. 2007), tumor growth and angiogenesis were examined in C57BL/6 mice implanted subcutaneously with B16F10 melanoma cells. The mice had access to regular drinking water and to 1 percent ethanol in their drinking water for 12 hours each per day for 4 weeks, with tumor cells being implanted during the second week of ethanol administration. Compared with animals who only drank water, those who had access to ethanol developed palpable tumors sooner and had 2.2 times greater tumor weights at the end of the study. Analysis of the tumors indicated an increase in VEGF mRNA and VEGF protein, as well as increased tumor angiogenesis. Moreover, another marker of angiogenesis, VEGF-R1 (Flt-1), also was found in a greater number of tumor cells and endothelial cells in the surrounding tissue from the ethanol group compared with the control group.
Wu and Pruett (1999) determined the effects of acute ethanol administration (5 or 6 g/kg body weight) given through a tube inserted into the mouth (i.e., by oral gavage) on melanoma metastasis. Ethanol administered to female B6C3F1 mice 1 hour before intravenous inoculation of B16F10 melanoma cells greatly increased lung metastasis. The number of metastases also was increased when administration of 6 g/kg ethanol occurred 4 or 10 hours after tumor inoculation but not when it occurred 6 hours before tumor inoculation. About half of the increase in lung tumor nodules seemed to be related to ethanol- induced corticosterone production in the adrenal glands. Experiments analyzing whether ethanol-related inhibition of some aspect of NK-cell function also might contribute to the increased metastasis produced found no indication of significantly reduced NK-cell numbers or activity. However, further studies directed at determining the role of NK cells in the acute ethanol-induced increase in melanoma lung metastasis showed that ethanol inhibited experimental induction of NK-cell activity (in response to poly I:C), resulting in approximately a twofold increase in lung tumor nodules compared with control mice (Wu and Pruett 1999). Additional mechanistic studies indicated that a single dose of ethanol increased corticosterone levels (Collier et al. 2000) and also suppressed experimental induction of mRNA for a variety of cytokines (i.e., interferon alpha [IFN-α], interferon beta [IFN-b], IFN-γ, IL-6, IL-12, and IL-15) (Pruett et al. 2003). As a result, concentrations of IFN-α and IL-12 in the blood were decreased. Conversely, ethanol greatly enhanced the levels of IL-10 in the blood. However, these changes in serum cytokines were not mediated by corticosterone. Overall, these studies suggest that the increase in melanoma lung nodule formation after acute ethanol administration is complex and related to effects associated with some aspect of NK-cell inhibition, increased suppression of NK-cell activity by corticosterone, and corticosterone-independent effects on cytokines favoring tumor immune escape.
The effects of chronic alcohol consumption on tumor growth and metastasis of the highly invasive and spontaneously metastatic B16BL6 melanoma inoculated subcutaneously were studied in female C57BL/6 mice administered ethanol in drinking water. In an initial study, consumption of 2.5 percent, 10 percent, or 20 percent w/v ethanol in drinking water for 6 to 8 weeks before tumor inoculation and continuing thereafter did not affect primary tumor growth (Blank and Meadows 1996). However, the animals receiving 20 percent ethanol in their drinking water exhibited consistently reduced survival, lower tumor weight, and lower final body weight compared with the other groups. Alcohol exposure also affected metastasis. All three ethanol-exposed groups had reduced metastasis to the axillary lymph nodes, with the 10-percent and 20-percent ethanol groups showing reduced lung metastasis, and the 20-percent ethanol group showing reduced superficial metastasis to the kidneys. Metastasis did occur, however, in the draining inguinal lymph nodes in mice consuming 20 percent weight per volume ethanol for 12 weeks (Zhang et al. 2011b).
More specific experiments sought to determine the effect in mice on metastasis of B16BL6 melanoma of short-term and long-term exposure to 10 percent and 20 percent w/v ethanol before and after intravenous inoculation into a tail vein or inoculation into the skin of the pinna of the ear2 (Meadows et al. 1993a,b; Spitzer et al. 2000; Zhang et al. 2011a). These experiments showed that 10 percent w/v ethanol did not affect metastasis after intravenous tumor inoculation in female C57BL/6 mice consuming alcohol for 2 weeks or spontaneous metastasis in mice injected 1 week after initiating ethanol feeding. However, lung metastasis was inhibited if intravenous injection of tumor cells occurred at 4, 6.5, 7, and 12 weeks after initiation of 20 percent w/v ethanol. Similarly, spontaneous metastasis to the lung was significantly inhibited in mice injected with melanoma at 1, 4, 6.5, and 10 weeks of consuming 20 percent ethanol. Ethanol did not prevent spontaneous metastasis to the draining cervical lymph nodes.
2 This method of tumor placement facilitates spontaneous metastasis.
The antimetastatic effect of ethanol on the B16BL6 melanoma tumor was confirmed after injection of tumor cells in male C57BL/6J mice that carry a gene for obesity and which had consumed 20 percent ethanol in drinking water for 10 weeks (Kushiro and Nunez 2012). Different groups were analyzed for lung tumor metastasis over a period of 16 to 21 days. All of the water-drinking animals had developed visible lung metastases at 16 days after tumor injection, whereas some of the ethanol-drinking mice did not develop lung metastases until 21 days. Moreover, the numbers of lung metastases in the ethanol-drinking mice were significantly lower at 21 days.
The contribution of NK cells to the inhibition of metastasis was evaluated in mice consuming 10 percent or 20 percent w/v ethanol for 4 weeks (Meadows et al. 1993a). Whereas consumption of 10 percent ethanol did not alter the NK cells’ ability to destroy other cells (i.e., decrease cytolytic activity), animals consuming 20 percent ethanol showed decreased NK cytolytic activity. And although experimental stimulation of NK cells could enhance their cytolytic activity 4.3-fold in the ethanol-drinking animals, compared with 2.6-fold in the control animals, overall cytolytic activity still was lower in the ethanol group than in the control group. Treatment of mice with an antibody against NK cells (i.e., anti-NK1.1 antibody) markedly decreased NK-cell cytolytic activity in both water- and ethanol-drinking animals. These same treatments were evaluated in mice injected with B16BL6 melanoma. Experimental stimulation of NK cells decreased the number of lung metastases in the water-drinking and 10-percent ethanol groups, but not in the 20-percent ethanol group. Inactivation of NK cells by administration of anti-NK1.1 antibody significantly increased lung metastases in the water-drinking and 10-percent ethanol groups, but not in the 20-percent ethanol group.
The data suggest that inhibition of NK-cell cytolytic activity in mice consuming 20 percent ethanol does not lead to enhanced metastasis following inoculation of B16BL6 melanoma. This lack of interaction between alcohol consumption and NK-cell cytolytic activity in B16BL6 melanoma lung metastasis was further confirmed in another strain of mice (i.e., beige mice) that naturally have low NK cytolytic activity (Spitzer et al. 2000). In other experiments to determine how ethanol decreases metastasis of B16BL6 melanoma, either isolated tumor cells grown in the presence of 0.3 percent ethanol or tumor cells from alcohol-consuming mice were inoculated into water-drinking mice (Blank and Meadows 1996). Mice that had received either tumor cells cultured in the presence of ethanol or derived from mice drinking 20 percent alcohol showed increased lung metastasis compared with control mice or those receiving tumor cells derived from mice drinking 10 percent ethanol. Thus, ethanol seems to increase the actual metastatic potential of melanoma cells. However, because alcohol drinking inhibits metastasis, there seem to be other factors induced by ethanol that counter this metastatic potential.
Further research was directed at identifying factors involved in expression of the antimetastatic effect associated with chronic (12 weeks) consumption of 20 percent w/v alcohol consumption. Zhang and colleagues (2011a) found that chronic alcohol consumption increased the numbers of immune cells (e.g., NK, NKT, CD4+ T and CD8+ T cells) that produce IFN-γ. IFN-γ is an essential factor in the control of melanoma metastasis (Blankenstein and Qin 2003; Ikeda et al. 2002). IFN-γ also mediates the antimetastatic effects of chronic alcohol consumption, because mice that could not produce IFN-γ did not show this effect (Zhang et al. 2011a). The investigators attempted to determine whether one immune cell population was more important than another in producing the antimetastatic IFN-γ. However, through a series of specific cell depletion experiments, they found that it did not matter which cell type produced the IFN-γ.
Other investigators (Kushiro and Nunez 2012) found that B16BL6 melanoma cells grown in 0.1 percent, 0.2 percent, or 0.5 percent ethanol showed considerably reduced cell invasion and, at the highest ethanol concentration, reduced cell motility and anchorage-dependent growth. Ethanol did not affect cell proliferation, apoptosis, or necrosis of the melanoma cells. In addition, the highest ethanol dose altered the expression of several genes that play prominent roles in regulating melanoma metastasis (i.e., the IL6, Nfkb, snail1, E-cadherin, Kiss1, Nm23-m1, and Nm23-m2 genes). These changes also could contribute to the antimetastatic effect of alcohol on melanoma.
Summary
Animal models have yielded some insights into the effects of alcohol on tumor growth, survival, and metastasis of different cancers, including breast cancer, lung cancer, liver cancer, and melanoma. However, because cancer is a collection of many different diseases and subtypes, each cancer or cancer subtype might not respond similarly to alcohol, as is evident from the research discussed here.
The relationship between alcohol and tumor progression is complex. Tumor metastasis involves many steps and also is controlled by many different signaling pathways. Because each cancer is unique, the specific connections between alcohol and the steps involved in cancer progression also by nature are complex. Thus, it is important to relate experimental results to specific types/subtypes of cancer as well as the amount of alcohol consumed, the route of alcohol administration, and the duration of alcohol administration. Clearly, more studies are needed to define the effect of alcohol on tumor progression and to determine the mechanisms associated with individual cancers and subtypes.
References
Aye, M.M.; Ma, C.; Lin, H.; et al. Ethanol-induced in vitro invasion of breast cancer cells: The contribution of MMP-2 by fibroblasts. International Journal of Cancer 112(5):738–746, 2004. PMID: 15386367
Ben-Eliyahu, A.; Page, G.G.; Yirmiya, R.; and Taylor, A.N. Acute alcohol intoxication suppresses natural killer cell activity and promotes tumor metastasis. Nature Medicine 2(4):457–460, 1996. PMID: 8597957
Blank, S.E., and Meadows, G.G. Ethanol modulates metastatic potential of B16BL6 melanoma and host responses. Alcoholism: Clinical and Experimental Research 20(4):624–628, 1996. PMID: 8800377
Blankenstein, T., and Qin, Z. The role of IFN-gamma in tumor transplantation immunity and inhibition of chemical carcinogenesis. Current Opinion in Immunology 15(2):148–154, 2003. PMID: 12633663
Capel, I.D.; Jenner, M.; Pinnock, M.H.; et al. The effect of chronic ethanol intake on the growth and spread of some murine tumors. Oncology 35(5): 224–226, 1978. PMID: 569263
Collier, S.D.; Wu, W.J.; and Pruett, S.B. Ethanol suppresses NK cell activation by polyinosinic-polycytidylic acid (poly I:C) in female B6C3F1 mice: Role of endogenous corticosterone. Alcoholism: Clinical and Experimental Research 24(3):291–299, 2000. PMID: 10776665
Gu, J.W.; Bailey, A.P.; Sartin, A.; et al. Ethanol stimulates tumor progression and expression of vascular endothelial growth factor in chick embryos. Cancer 103(2):422–431, 2005. PMID: 15597382
Hoefnagel, L.D.; van de Vijver, M.J.; van Slooten, H.J.; et al. Receptor conversion in distant breast cancer metastases. Breast Cancer Research 12(5):R75, 2010. PMID: 20863372
Holcomb, V.B.; Hong, J.; and Nunez, N.P. Exogenous estrogen protects mice from the consequences of obesity and alcohol. Menopause 19(6):680–690, 2012. PMID: 22228320
Hong, J.; Holcomb, V.B.; Kushiro, K.; and Nunez, N.P. Estrogen inhibits the effects of obesity and alcohol on mammary tumors and fatty liver. International Journal of Oncology 39(6):1443–1453, 2011. PMID: 21850368
Hong, J.; Holcomb, V.B.; Tekle, S.A.; et al. Alcohol consumption promotes mammary tumor growth and insulin sensitivity. Cancer Letters 294(2):229–235, 2010. PMID: 20202743
Ikeda, H.; Old, L.J.; and Schreiber, R.D. The roles of IFN gamma in protection against tumor development and cancer immunoediting. Cytokine & Growth Factor Reviews 13(2):95–109, 2002. PMID: 11900986
Ketcham, A.S.; Wexler, H.; and Mantel, N. Effects of alcohol in mouse neoplasia. Cancer Research 23:667–670, 1963. PMID: 14032175
Kudo-Saito, C.; Shirako, H.; Ohike, M.; et al. CCL2 is critical for immunosuppression to promote cancer metastasis. Clinical & Experimental Metastasis 30(4):393–405, 2013. PMID: 23143679
Kushiro, K., and Nunez, N.P. Ethanol inhibits B16-BL6 melanoma metastasis and cell phenotypes associated with metastasis. In Vivo 26(1):47–58, 2012. PMID: 22210715
Lu, Y.; Ni, F.; Xu, M.; et al. Alcohol promotes mammary tumor growth through activation of VEGF-dependent tumor angiogenesis. Oncology Letters 8(2):673–678, 2014. PMID: 25009649
Luo, J., and Miller, M.W. Ethanol enhances erbB-mediated migration of human breast cancer cells in culture. Breast Cancer Research and Treatment 63(1):61–69, 2000. PMID: 11079160
Ma, C.; Lin, H.; Leonard, S.S.; et al. Overexpression of ErbB2 enhances ethanol-stimulated intracellular signaling and invasion of human mammary epithelial and breast cancer cells in vitro. Oncogene 22(34): 5281–5290, 2003. PMID: 12917629
Meadows, G.G.; Elstad, C.A.; Blank, S.E.; et al. Alcohol consumption suppresses metastasis of B16-BL6 melanoma in mice. Clinical & Experimental Metastasis 11(2):191–199, 1993a. PMID: 8444011
Meadows, G.G.; Elstad, C.A.; Blank, S.E.; et al. Ethanol and melanoma metastasis. In: Advances in the Biosciences. Alcohol, Drugs of Abuse, and Immunomodulation (AIDS): Proceedings of the 2nd International Conference on Alcohol, Drugs of Abuse and Immunomodulation (AIDS) Held in Arizona, USA, on 9–13 September 1992. Vol. 86. London: Pergamon Press, 1993b, pp. 399–410.
Pruett, S.B.; Fan, R.; and Zheng, Q. Acute ethanol administration profoundly alters poly I:C-induced cytokine expression in mice by a mechanism that is not dependent on corticosterone. Life Sciences 72(16):1825–1839, 2003. PMID: 12586220
Ranuncolo, S.M.; Matos, E.; Loria, D.; et al. Circulating 92-kilodalton matrix metalloproteinase (MMP-9) activity is enhanced in the euglobulin plasma fraction of head and neck squamous cell carcinoma. Cancer 94(5):1483–1491, 2002. PMID: 11920505
Sanchez-Alvarez, R.; Martinez-Outschoorn, U.E.; Lin, Z.; et al. Ethanol exposure induces the cancer-associated fibroblast phenotype and lethal tumor metabolism: Implications for breast cancer prevention. Cell Cycle 12(2):289–301, 2013. PMID: 23257780
Sarvaiya, P.J.; Guo, D.; Ulasov, I.; et al. Chemokines in tumor progression and metastasis. Oncotarget 4(12):2171–2185, 2013. PMID: 24259307
Singletary, K.W.; Frey, R.S.; and Yan, W. Effect of ethanol on proliferation and estrogen receptor-alpha expression in human breast cancer cells. Cancer Letters 165(2):131–137, 2001. PMID: 11275361
Spitzer, J.H.; Nunez, N.P.; Meadows, S.A.; et al. The modulation of B16BL6 melanoma metastasis is not directly mediated by cytolytic activity of natural killer cells in alcohol-consuming mice. Alcoholism: Clinical and Experimental Research 24(6):837–844, 2000. PMID: 10888072
Tan, W,; Bailey, A.P.; Shparago, M.; et al. Chronic alcohol consumption stimulates VEGF expression, tumor angiogenesis and progression of melanoma in mice. Cancer Biology & Therapy 6(8):1211–1217, 2007. PMID: 17660711
Thompson, K.J.; Swan, R.Z.; Iannitti, D.A.; et al. Diet-induced obesity and ethanol impair progression of hepatocellular carcinoma in a mouse mesenteric vein injection model. Surgical Endoscopy 27(1):246–255, 2013. PMID: 22806512
Vorderstrasse, B.A.; Wang, T.; Myers, A.K.; et al. Alcohol consumption suppresses mammary tumor metastasis in a syngeneic tumor transplantation model. Breast Cancer Research and Treatment 136(3):729–737, 2012. PMID: 23117853
Wang, S.; Xu, M.; Li, F.; et al. Ethanol promotes mammary tumor growth and angiogenesis: The involvement of chemoattractant factor MCP-1. Breast Cancer Research and Treatment 133(3):1037–1048, 2012. PMID: 22160640
Wong, A.W.; Paulson, Q.X.; Hong, J.; et al. Alcohol promotes breast cancer cell invasion by regulating the Nm23-ITGA5 pathway. Journal of Experimental & Clinical Cancer Research 30:75, 2011. PMID: 21838876
Wu, W.J., and Pruett, S.B. Ethanol decreases host resistance to pulmonary metastases in a mouse model: Role of natural killer cells and the ethanol-induced stress response. International Journal of Cancer 82(6):886–892, 1999. PMID: 10446458
Xu, M.; Bower, K.A.; Wang, S.; et al. Cyanidin-3-glucoside inhibits ethanol-induced invasion of breast cancer cells overexpressing ErbB2. Molecular Cancer 9:285, 2010. PMID: 21034468
Yirmiya, R.; Ben-Eliyahu, S.; Gale, R.P.; et al. Ethanol increases tumor progression in rats: Possible involvement of natural killer cells. Brain, Behavior, and Immunity 6(1):74–86, 1992. PMID: 1571604
Zhang, H.; Zhu, Z.; McKinley, J.M.; and Meadows, G.G. IFN-gamma is essential for the inhibition of B16BL6 melanoma lung metastasis in chronic alcohol drinking mice. Clinical & Experimental Metastasis 28(3):301–307, 2011a. PMID: 21234656
Zhang, H.; Zhu, Z.; and Meadows, G.G. Chronic alcohol consumption decreases the percentage and number of NK cells in the peripheral lymph nodes and exacerbates B16BL6 melanoma metastasis into the draining lymph nodes. Cell Immunology 266(2):172–179, 2011b. PMID: 20974468
Zhu, Y.; Lin, H.; Li, Z.; et al. Modulation of expression of ribosomal protein L7a (rpL7a) by ethanol in human breast cancer cells. Breast Cancer Research and Treatment 69(1):29–38, 2001. PMID: 11759826
Acknowledgments
Funding for this research was provided by NIAAA grants K05–AA–017149 to Dr. Meadows and R21–AA–022098 to Drs. Meadows and Zhang.
Disclosures
The authors declare that they have no competing financial interests.